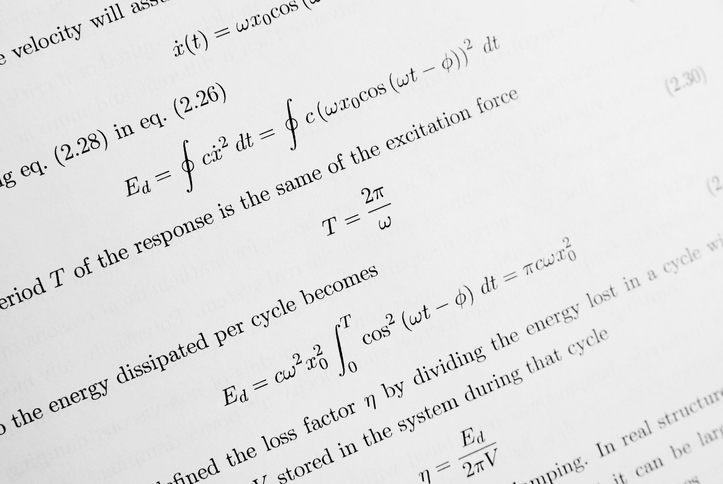
Misconceptions about Quantum Physics
If you put the word “quantum” in a scientific talk title, you immediately lose the interest of 90% of your non-physics audience. But if you put the same word in a public lecture title, the interest level increases tenfold.
What is it about quantum physics that captures the public fascination—yet scares off many scientists and engineers—more than just about any other subject? Is quantum physics somehow fundamentally “different” from all other areas of scientific inquiry? Nearly one hundred years after its discovery, the “true meaning” of the quantum theory remains as elusive as ever—even as it continues to make the most accurate experimental predictions in all of science.
But despite genuine controversy, needless misconceptions also abound.
This presentation will ground the discussion by addressing what the basic experimental facts imply about the nature of: (1) nonlocality; (2) the quantum wavefunction; (3) what can be measured. Only then will more esoteric aspects be discussed, e.g.: What role, if any, do conscious observers play in determining quantum reality? Do “many quantum worlds” really exist, and if so where are they exactly? What does quantum physics have to do with extra dimensions, teleportation, eastern mysticism, etc.?
Einstein’s Biggest Blunder? A Cosmic Mystery Story
In 1915 Einstein completed his greatest triumph, the General Theory of Relativity.
This remarkable theory laid the basis not just for our understanding of the motion of objects within the Universe, but the motion of the universe itself! Yet, in 1916, it looked as if Einstein’s theory did not properly account for observations of the universe on large scales. To resolve this problem, he added an additional term to his equations, the so-called “Cosmological Constant”. Within a decade however, observations indicated that such a term was not necessary to obtain agreement with observations, and Einstein called this addition his “biggest blunder”.
Over the past decade, new observations have led to a revolution in cosmology. The standard model of cosmology built up over a 20 year period up until the early 1990’s is now dead. Its replacement may be far more bizarre. In particular, new data from a wide variety of independent cosmological and astrophysical observations, combine together to strongly suggest most of the energy density of the universe today may be contained in empty space! Remarkably, this is exactly what one would expect if Einstein’s Cosmological Constant really exists! If it does, its origin is the biggest mystery in physics. The discussion will end by briefly describing possible implications for our understanding of nature, for physics, and for life, of this astounding new result.
Extra Dimensions
There has been a revolution in our thinking about extra dimensions. A new understanding of the feasibility of localizing four dimensional gauge theories in higher dimensional spacetimes has led to a variety of phenomenologically viable models, and even to the possibility of localizing gravity. Unlike older theories of extra dimensions, much of the focus now is on extra dimensions with sizes on the order of one thousandth of a proton width or larger! Thus, there is a potential for discovery at current and soon-to-be-completed colliders, and in some cases table-top experiments. In addition there are tremendous implications for cosmology. The following topics will be covered:
- Einstein and the Fourth Dimension
- Waves in a Fifth Dimension
- String Theory and Branes
- Experimental Tests of Extra Dimensions
Finding a Home in the Multiverse
The history of cosmology over the past millennium has been that of an ever-widening view of the universe, from Earth surrounded by planets and “crystal spheres” of stars, to a solar system surrounded by thousands of distant stars, then to millions of stars, then to the realization that these millions comprise but one galaxy of millions in the observable universe. Over the past 20 years, several lines of thought have emerged suggesting that the universe may, in fact, be vastly larger yet — it may in fact be a “Multiverse” of uncountable regions as large as all that we can observe, yet potentially with very different properties.
Several lines of reasoning will be discussed — from quantum mechanics, string theory, and observational and theoretical cosmology — that both individually and in conjunction lead us to suspect that we may inhabit some sort of multiverse. Also, the following questions will be addressed: How likely are these different scenarios? How do we describe a mulitverse and what is its structure? Are other universes observable? Are multiverse theories testable, and how can they be tested if we only observe one universe?
INTO THE DARK: The Future History of the Universe
This talk outlines the long term fate of the cosmos. We consider the
evolution of planets, stars, galaxies, and the universe itself over time scales that greatly exceed the current age of the universe. This discussion starts with new stellar evolution calculations that follow the development of the low mass (M type) stars that dominate the stellar IMF. We then determine the final mass distribution of stellar remnants – the neutron stars, white dwarfs, and brown dwarfs remaining at the end of stellar evolution. After several trillion years, the supply of interstellar gas grows depleted, yet star formation continues at a highly attenuated rate through brown dwarf collisions. This process tails off as the galaxy gradually loses its stars by ejecting the majority, and driving a minority toward accretion onto massive black holes. As the galaxy disperses, weakly interacting dark matter particles are accreted by white dwarfs, where they subsequently annihilate and keep the old stellar remnants relatively “warm”. After accounting for the destruction of the galaxy, we consider the fate of expelled degenerate objects (planets, white dwarfs, and neutron stars) within the assumption that proton decay is a viable process. The evolution and eventual sublimation of these objects is dictated by the decay of their constituent nucleons; this scenario is developed in some detail. After white dwarfs and neutron stars have disappeared, the black holes slowly lose their mass as they emit Hawking radiation. After the largest black holes have evaporated, the universe slowly slides into darkness.
Is time travel possible?
Einstein’s General Relativity tells us that space and time together form a 4-dimensional spacetime that is curved by the presence of matter and energy. If we could produce the proper state of matter and energy, the spacetime could curve enough to permit travel into the past. But ordinary forms of matter are not sufficient. Instead we
would need exotic material with negative energy density. The possibility of time travel depends on whether quantum mechanics can provide us with the proper negative-energy-density state.
The lecture will present the state of the art in designing a time machine or proving that it is impossible to do so, and the related issues of wormholes and faster-than-light travel. Time-travel ideas related to quantum mechanical correlations and tunneling of a signal through a barrier will be briefly discussed.
Misconceptions about Particles and Space-Time
With the excitement of the discovery of the Higgs boson at the Large Hadron Collider also came troubling headlines that the collider could also produce black holes that would destroy the earth. Other headlines claimed that the collider could prove the existence of Star Trek’s parallel universe by sending particles into extra dimensions. There have also been renewed claims made for the existence of cold fusion, which if true could dramatically change our world. The science behind these stories and others will be examined and clarified.
Multiverse—A Revolution in Modern Cosmology
Recent observational and theoretical discoveries have led to the picture that what we thought of as the entire universe is only a tiny part of a much larger structure, often called the “multiverse.” In the multiverse, there are infinitely many universes in which what we once thought were fundamental laws of physics may take different forms than those in our universe; for example, they may have different elementary particles and even different spacetime dimensions. This picture has led to some controversies. In particular, it has led to the question: is this really science? I argue that multiverse cosmology follows precisely the standard methodologies in science: forming a hypothesis about the natural world based on observation (with the help of mathematics) and then looking for evidence that further supports it. Several misconceptions associated with multiverse cosmology will be discussed. These include statements such as “it allows us to contemplate anything so there is no implication” and “it is not falsifiable”—none of these statements is true. In fact, it rather stimulates new experimental probes that might reveal further evidence for the paradigm. I also discuss the possibility that the multiverse picture helps us to understand deep structures of a quantum theory of gravity and spacetime, which has so far been elusive.
Our Miserable Future
Discoveries in cosmology over the past decade have revolutionized our view of the future of the universe and life within it. These new discoveries and their implications for the future of life will be discussed. The focus will be on our own future in the relatively near term (i.e. the next 5 billion years), which will provide challenges for the future of intelligent life in our solar system, and then moving on to the very far future, when it appears the universe we live in may be worst of all universes for the future of life. Even before life itself ends, as it must in such a universe, the quality of life will decrease, as our empirical knowledge of the universe will also decrease. All evidence of the big bang, and of the existence of other galaxies outside our own will disappear on a timeframe that is short compared to the lifetime of the longest lived stars. Astronomers in the future will believe they live in a static universe with only one island galaxy. Remarkably the current epoch seems quite special in the history of the universe for a variety of reasons. Is there any significance to this? Arguments about this are changing the way many scientists view a possible understanding of nature.
String Theory: A Theory of Anything? A Theory of Nothing? A Theory?
The long-standing fascination that physicists, as well as artists and writers, have had for the idea that there are more dimensions to the universe than meets the eye, will be discussed. The discussion continues with, how modern research in string theory has evolved from a long history of extrapolating from real physical problems to abstract solutions that may involve new, hidden physics, and possible hidden new dimensions to the universe. In the process the idea for string theory arose, but it has really not progressed far beyond the ‘hope’ for a theory in the past 25 years. Moreover, this ‘hoped-for’ theory has morphed from a possible “theory of everything” in the 1980’s to a possible “theory of anything” in the past decade. Whether this new focus on the question of whether the laws of nature represent a mere environmental accident,–via something called the anthropic principle–or whether they are based on fundamental immutable principles will represent the end of fundamental knowledge remains to be seen.
String Theory: What is it Good for?
The beginnings of string theory as an attempt to understand strongly bound quarks will be reviewed. How string theory evolved into a theory of quantum gravity will also be covered before moving on to more recent developments including the Maldacena conjecture and it’s applications to heavy ion collisions, the five dimensional Randall-Sundrum model, and a possible origin for the electron mass.
The Beginning and End of Time: Life, The Universe, and Nothing
One can consider measuring time by the number of events that occur within some period. In this sense, more happened in the first second in the history of the universe than has occurred in the history of the universe since that moment. The first part of the lecture highlights some of the major milestones in that initial moment, and then moves on to discuss the future. Our current observations suggest we live in the worst of all universes for the long term future of life, and that our knowledge about the state of the universe will continue to decrease with time. In the far future we will be alone in a largely dark and empty universe.
The Nature of Space and Time
In honor of the hundredth anniversary of Einstein’s “miraculous year” of 1905, the modern view of space and time will be discussed. The discussion starts with special relativity and then follows by how space and time are modified in Einstein’s general theory of relativity. The discussion ends with some recent ideas including black holes in higher dimensions. In all cases, the view of space and time arising from modern physics is radically different from our everyday experience, yet many of their strange properties have already been confirmed by experiment
Two-Time Physics: The Unified View From Higher Dimensional Space and Time
Evidence has been accumulating that the ordinary formulation of physics, in a space-time with three space and one time dimensions, is insufficient to describe our world, just like shadows on walls alone are insufficient to capture the true essence of an object in a three dimensional room. Two-Time Physics reveals that our physical world in 3+1 dimensions is like a shadow of a highly symmetric universe in four space and two time dimensions. Amazingly, the best understood fundamental theory in Physics, the Standard Model of Particles and Forces is reproduced, and its “strong CP problem” is solved, as a field theory in 4+2 dimensions in the context of Two-Time Physics. This point of view provides new mathematical tools and new insights for understanding our universe. Evidence of the 4+2 dimensional world can be found both at the macroscopic and microscopic scales in the form of hidden symmetries and “dualities”, and such predictions of Two-Time Physics can be tested through theory and experiment. Two-Time Physics may assist in the quest to unify the Standard Model with Einstein’s theory of General Relativity in a single unified theory. The most popular approach to that problem today, superstring theory, and its extension M theory, invoke 10 dimensions of space, but a single dimension of time. The path to success with formulating M theory, which so far eluded theoretical physicists, could well be adopting the more symmetric and higher dimensional Two-Time Physics approach. This would require adding one time dimension plus one space dimension, giving nature 11 space and two time dimensions. The Two-Time Physics version of M theory would have a total of 13 dimensions.
The World as a Hologram
Physicists have come to recognize an unexpected, very general relation between Einstein’s curved geometry of space and time, and the amount of information required to describe physical matter down to the smallest particles. This “holographic principle” is set to play a crucial role as we tackle the profound problem of unifying quantum mechanics with gravity. The session begins with the discussion of some everyday ways in which matter contains information, and how we can describe the state of matter by listing that information. It will be then explained what we have learned about the information content of black- holes, and how this knowledge has allowed us a first glimpse at the truly universal limits on information content that have since been discovered. Professor Bousso will emphasize in particular the counterintuitive nature of these limits: they imply that in a sense, the universe is only pretending to have 3 spatial dimensions, like a two-dimensional hologram on a credit card.